Dr Kirsty Leśniak SFHEA, PGCE, MSc, BSc (Hons), explains her research into the impact of the shoeing cycle on long term soundness
The importance of a regular shoeing cycle is undisputed. However, regardless to the placement of the shoe, the regularity of the intervention can be the deciding factor as to the success of the hoof care effort and, longer term soundness.
What is the ideal?
Regularity should take in to account the length of time between visits from the farrier, not just that those intervals are constant over a defined time period. For instance, a repeated nine-week interval could be considered regular but, the duration between the intervals is in no way ideal for the horse’s long-term soundness. Factors influencing the duration between visits from the farrier include, horn quality, performance discipline, general health status, climate, ground conditions, nutrition, progress of remedial farriery treatment and durability of materials used, amongst others.
The cost factor
However, financial constraints of the owner often influence the duration between interventions, with little understanding of the potential effects on the soundness of the horse. The generally recommended interval is 4 to 6 weeks¹ ².
Nevertheless, when the average cost for a set of shoes is approximately £90, a slight lengthening of intervals between interventions would result in significant annual savings. Even working to a six-week rather than four-week interval results in four fewer farrier visits per year; an annual saving of approximately £350.
Pushing the interval to seven weeks can save approximately £450 annually and a routine eight-week interval could halve the annual financial outlay. The appeal to owners is therefore easy to understand, especially for those facing other financial pressures. While increasing the interval duration is unlikely to have a detrimental impact in the short-term, repeated use has the potential for much greater impacts on the horse’s health.
What is the hoof pastern axis and why is it important?
With 60% of the horse’s mass transmitted through the forelimbs of the standing horse, the structural conformation of the limb is fundamental in enabling them to sustain the load they have to support. Research has evidenced a relationship between the forces through the limb and the limb orientation, that results from hoof conformation and resultant hoof pastern axis (HPA)³. It’s therefore the role of the farrier to ensure that, through appropriate hoof conformation and foot balance, the correct HPA, and consequentially limb orientation, is maintained.
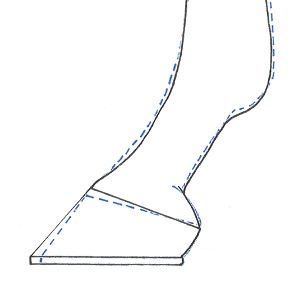
When viewed laterally, the vertical pillar of support provided by the forelimb structure terminates at the metacarpophalangeal (MCP) joint.The MCP joint angulation requires that loading forces through the limb be transmitted through both the bony column, and through the palmarly positioned soft tissue sling; collectively known as the suspensory apparatus.
The collation of structures forming the suspensory apparatus includes the superficial digital flexor tendon (SDFT), the deep digital flexor tendon (DDFT), the suspensory ligament (SL), and the sesamoidean, collateral and annular ligaments.
While the ligaments secure and stabilise the joints, the SDFT and DDFT act both to shock absorb, thereby further stabilising the joints and, by functioning as energy efficient springs during locomotion. While research has not yet identified the exact loading ratios between the bony column and the suspensory apparatus, what is considered an ideal angulation for the HPA has been widely documented. HPA angulations have been reported within research to range between 42° to 58°, with mean values ranging from 51.8° and 53.7° ⁴ ⁵. However, consensus agrees that a truly appropriate angle is one that remains continuous with the alignment of the pasterns.
Changes to this angulation affect the dorsopalmer distribution of the load across the hoof. When the dorsal hoof wall angle (DHWA) is angled at 55˚, approximately 57% of the loading weight is transmitted to the heel region. This loading increases to 75% with a 16˚ decrease to a DHWA angle to 39˚⁶. The load placed on the suspensory apparatus, and therefore the loading on the mechanical springs, is proportional to the slope of the hoof-pastern axis.
The natural interaction between the hoof capsule and the ground in barefoot horses, enables a wear distribution that mitigates significant angular and linear changes in hoof geometry between interventions⁷. Placement of a shoe protects the toe from the frictional wear that results from ground contact. Albeit reduced, heel expansion is still permitted at the quarters and heel of shod horses, facilitating a small amount of wear⁸. As a result, the length of the toe increases between interventions faster than heel length. The disproportionate wear between the toe and the heel results in a decreasing DHWA and consequentially, the HPA progressively takes on a more broken back alignment.
The DIP joint plays a vital role in the function of the horse’s foot and alterations in the orientation of this joint are normally aimed at compensating for changes in gradient or direction⁹ . The more extended distal limb posture, particularly of at the level of the distal interphalangeal joint (DIP) results in a 3-5˚ decrease in the angulation of the distal phalanx (P3) within the standing posture¹. Despite the close relationship between the DIP and proximal interphalangeal joints (PIP), PIP joint angulation does not significantly alter across the intervention interval⁹; this is likely due to its extensive ligamentous support.
This more horizontal alignment of P3 further increases tension on the DDFT, which is trying to oppose the forces resulting from P3 to maintain a dynamic equilibrium between the interacting structures¹⁹¹⁰. Prior to midstance of the stride, the DIP joint is in flexion but, as the body mass moves over the top of the limb during the stance phase it moves into extension⁹.
In the standing horse, a broken-back HPA forces the joint to more extended posture, therefore during the stride it would result in a greater range of flexion pre-midstance, through an increase in the moment arm¹ ¹¹ ¹² Despite its minimal range of movement, the PIP joint does demonstrate compensatory extension alongside the MCP joint under high loading¹³. The resultant extended posture alters the joint surface alignment and, can result in interference of extensor process of P3 with the dorsal articular border of P2, and the dorsal articular borders of the MCP joint, potentially leading to osteolytic changes.
What does the science show?
Many publications investigating dorsopalmar imbalances have attained their results either through application of wedges¹⁴, or through use of eight to ten-week intervention intervals¹⁵. Unlike the graduated soft tissue response, which would be expected over an intervention interval, the application of artificial wedges results in an immediate and radical alteration to the limb alignment. An eight to ten-week intervention interval is however, almost double that normally advised and changes to hoof geometry would therefore, well exceed those observed within routine practice. As such, neither scenario extrapolates well into practice.
The focus of our 2017 paper published in Animals², was therefore to review similar morphometric parameters as previously considered, but within the more practicably applicably four to six week intervention period. The results highlighted an increase in the dorsal hoof wall angle of 2.3˚ following farriery intervention after a four to six-week interval; an amount reflective of the decrease in DHWA between interventions. If considered as change in angulation per week, these findings were consistent with those previously reported for an eight to ten week interval ¹. The intervention significantly reduced DHW length, though less than expected likely due to the normal decrease in hoof growth at the time of year during which data was collected (November – January).
Through the reduction in DHW length a significant correction of the phalangeal alignment occurred as a result of the dorsal rotation of the hoof position and repositioning of the DIP joint. Furthermore, the change in orientation of the foot across the four to six-week intervention interval, led to a significant palmar shift in the centre of pressure of the foot. Consequentially, the position of the vertical limb column also moved palmarly. This palmar shift alters the load ratio between the hard and soft tissue structures with the resulting posture inhibiting the heels from providing effective support for the loaded limb. The proportion of the load supported through the limb’s bony construct decreases, while that distributed to the suspensory apparatus increases, increasing risk of injury.
Figure 2: Rotation of DIP joint through loading of the joint. Red arrow: Rotation of the DIP joint through the pull of the DDFT a) Post trimming alignment; HPA parallel with DHWA, bodyweight supported by heels b) Pre-trimming after four to six-week interval; broken-back HPA, bodyweight not supported by heels.
Longer durations between hoof care interventions would result in more acutely DHWA and greater palmar loading. Similarly to bone, horn tubule strength comes from their ability to cope with compressive loads along the length of their axis¹⁶. Their ability to cope with such loads therefore relies on a relatively vertical orientation, enabling them to act as pillars of support. The more acute angulation of both the heel and DHW towards the end of a hoof care interval results in the load intersecting the axis along its length. The change in angulation exhibited by the heel (4.5˚) was almost twice that of the DHW (2.3˚).
The more acutely angled tubules of the heels make the thinner and weaker stratum medium of this region more susceptible to collapse due to the inability of to provide appropriate levels of support for an increasing palmar load. This scenario will be exacerbated as the duration between interventions increases; potentially resulting in the need for corrective, rather than routine farriery. With the potential for osteolytic changes, damage to the suspensory apparatus and heel collapse, the benefits of a regular four to six-week shoeing interval well exceed the savings made by elongating the intervals between hoof care interventions.
References
- Moleman M, van Heel MC V., van Weeren PR, Back W. Hoof growth between two shoeing sessions leads to a substantial increase of the moment about the distal, but not the proximal, interphalangeal joint. Equine Vet J. 2006;38(2):170–4.
- Leśniak K, Williams J, Kuznik K, Douglas P. Does a 4–6 week shoeing interval promote optimal foot balance in the working equine? Animals. 2017;7(4).
- Eliashar E, McGuigan MP, Wilson AM. Relationship of foot conformation and force applied to the navicular bone of sound horses at the trot. Equine Vet J [Internet]. 2004 Jan 5 [cited 2018 Feb 16];36(5):431–5. Available from: http://doi.wiley.com/10.2746/0425164044868378
- Clayton H. The effect of an acute hoof wall angulation on the stride kinematics of trotting horses. Equine Vet J [Internet]. 1990 Jun 10 [cited 2015 Nov 4];22(S9):86–90. Available from: http://doi.wiley.com/10.1111/j.2042-3306.1990.tb04742.x
- \McClinchey HL, Thomason JJ, Jofriet JC. Isolating the effects of equine hoof shape measurements on capsule strain with finite element analysis. Vet Comp Orthop Traumatol [Internet]. 2003 [cited 2019 Oct 17];16(02):67–75. Available from: https://www.thieme-connect.com/products/ejournals/html/10.1055/s-0038-1632762
- Barrey E. Investigation of the vertical hoof force distribution in the equine forelimb with an instrumented horseboot. Equine Vet J Suppl. 1990;9:35–8.
- Clayton HM, Gray S, Kaiser LJ, Bowker RM. Effects of barefoot trimming on hoof morphology. Aust Vet J [Internet]. 2011 Aug [cited 2016 Jan 18];89(8):305–11. Available from: http://www.ncbi.nlm.nih.gov/pubmed/24635632
- Brunsting J, Dumoulin M, Oosterlinck M, Haspeslagh M, Lefère L, Pille F. Can the hoof be shod without limiting the heel movement? A comparative study between barefoot, shoeing with conventional shoes and a split-toe shoe. Vet J. 2019 Apr 1;246:7–11.
- Clayton HM. Biomechanics of the Distal Interphalangeal Joint. J Equine Vet Sci [Internet]. 2010 Aug 1 [cited 2018 May 25];30(8):401–5. Available from: http://linkinghub.elsevier.com/retrieve/pii/S073708061000314X
- Page BT, Hagen TL. Breakover of the hoof and its effect on stuctures and forces within the foot. J Equine Vet Sci [Internet]. 2002 Jun [cited 2016 Feb 8];22(6):258–64. Available from: http://www.sciencedirect.com/science/article/pii/S0737080602700622
- Thompson KN, Cheung TK, Silverman M. The effect of toe angle on tendon, ligament and hoof wall strains in vitro. J Equine Vet Sci [Internet]. 1993 Nov [cited 2015 Nov 4];13(11):651–4. Available from: http://www.sciencedirect.com/science/article/pii/S0737080607803972
- Balch OK, Butler D, Collier MA. Balancing the normal foot: hoof preparation, shoe fit and shoe modification in the performance horse. Equine Vet Educ [Internet]. 1997 Jun [cited 2015 Nov 4];9(3):143–54. Available from: http://doi.wiley.com/10.1111/j.2042-3292.1997.tb01295.x
- Clayton HM, Sha DH, Stick JA, Robinson P. 3D kinematics of the interphalangeal joints in the forelimb of walking and trotting horses. Vet Comp Orthop Traumatol [Internet]. 2007 [cited 2018 Jun 14];20(1):1–7. Available from: http://www.ncbi.nlm.nih.gov/pubmed/17364088
- Chateau H, Degueurce C, Denoix J-M. Three-dimensional kinematics of the distal forelimb in horses trotting on a treadmill and effects of elevation of heel and toe. Equine Vet J [Internet]. 2006 Jan 5 [cited 2019 Dec 27];38(2):164–9. Available from: http://doi.wiley.com/10.2746/042516406776563260
- Kummer M, Geyer H, Imboden I, Auer J, Lischer C. The effect of hoof trimming on radiographic measurements of the front feet of normal Warmblood horses. Vet J [Internet]. 2006 Jul [cited 2015 Sep 22];172(1):58–66. Available from: http://www.sciencedirect.com/science/article/pii/S109002330500078X
- Kasapi MA, Gosline JM. Strain-rate-dependent mechanical properties of the equine hoof wall. J Exp Biol. 1996;199(5):1133–46